Fruit fly's complex symphony of vision
A neuronal microcircuit leverages the same type of signal for multiple purposes
An orchestra of complex neuronal networks performs a symphonic masterpiece called vision – an exciting field for neurobiologists like Alexander Borst, director at the Max Planck Institute for Biological Intelligence. In his department’s latest study, the scientists found a microcircuit which inverts excitatory to inhibitory signals and is thus able to transform a single type of neuronal input for multiple purposes. The discovery of this microcircuit is an important puzzle piece for the better understanding of the visual process of the fruit flies Drosophila and ultimately of vision itself.
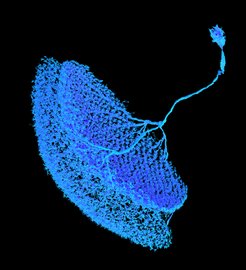
Vision is one of the most important senses in humans. Accordingly, a large part of the brain is dedicated to processing visual information. In order to compute visual information quickly and accurately, a multitude of neuronal networks must perform a complex interplay - which fruit flies can help us to understand. Surprisingly, besides the obvious differences between the eyes of humans and fruit flies, many parallels can be found in the way how their brains process visual information. Since the visual system of flies is very efficient but significantly less complex than ours, it's not surprising that this is one of the best understood neuronal network in neuroscience.
In the flies’ visual system, a cascade of cells is responsible to transform light information into direction-specific signals. T4 cells, for example, respond to moving bright edges (ON-pathway), while T5 cells only respond to moving dark edges (OFF-pathway). Both, T4 and T5 cells, have four subtypes that are tuned to the four cardinal directions (front-to-back, back-to-front, upwards and downwards). This means that each neuron only reacts to a specific direction of visual motion, their so-called preferred direction, while showing little reaction when stimulated by a moving edge in other directions (= null direction).
The mechanism to calculate direction selectivity in the ON-pathway has recently been deciphered by the department, leaving the exact implementation in the OFF-pathway yet to be understood. To this end, the scientists from Alexander Borst’s department developed a unique combination of calcium imaging with thermogenetic, optogenetic and pharmacologic techniques, to investigate the neuronal network underlying the OFF-pathway of Drosophila.
They found that several input neurons activate T5 cells when a signal moving in T5’s preferred direction is received. By suppressing or activating these input neurons, the scientists tested their role on how T5 cells compute direction. Taken together all the experiments, the team was able to confirm that the input neurons influence and shape the tuning of the T5 cells’ preferred direction.
In addition to activating input neurons, there are also inhibitory neurons, which reduce the outgoing signals of another cell. This is how all signals from directions other than the preferred direction is suppressed in T4 cells. Surprisingly, there are no such inhibitory neurons for T5 cells. This posed a mystery to the researchers: How does null direction suppression work in T5? In other words, how are these neurons able to react only to signals in their preferred direction without the inhibitory neurons?
The team lead by Amalia Braun discovered a microcircuit underlying the T5 null direction suppression: CT1, an unusual large-field neuron, receives and inverses excitatory inputs, which leads to an inhibitory signal needed for null direction suppression. “By using genetic methods to remove single cell types, we could show that the tuning curve of a T5 cell’s direction detection substantially broadened when CT1 is gone. This could also be confirmed by using algorithmic modelling”, explains Amalia Braun. “Thus, while the excitatory neurons influence T5 cells directly for their preferred direction, an excitatory signal taking the detour via a CT1 cell inverting the signal leads to null direction suppression.”
With the discovery of this microcircuit, the department could once again add a new act to the complex symphonic composition of Drosophila’s visual system. “The CT1 microcircuit is a striking example for the multilayered and diverse mechanisms of inhibition and excitation in the brain, and how a neuronal network leverages a single type of signal for multiple purposes”, summarizes last author Matthias Meier.
The identification of this microcircuit, enabling and shaping motion detection, is substantially deepening our understanding of the visual processing of Drosophila – and ultimately of vision itself.