Cell-cell communication in the developing brain
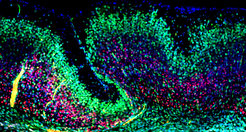
The folding of the mammalian cerebral cortex into sulci and gyri is thought to be favored by the amplification of basal progenitor cells and their tangential migration. We have provided a molecular mechanism for the role of migration in this process by showing that changes in intercellular adhesion of migrating cortical neurons result in cortical folding. Mice with deletions of FLRT1 and FLRT3 adhesion molecules develop macroscopic sulci with preserved layered organization and radial glial morphology. Cortex folding in these mutants does not require progenitor cell amplification, but is dependent on changes in neuron migration. Analyses and computer simulations suggest that sulci formation in the absence of FLRT1/3 results from reduced intercellular adhesion, increased neuron migration speed and neuron clustering in the cortical plate (Del Toro, Ruff, et al., 2017). nervous system and resulting changes in circuit development and animal behavior are being investigated.
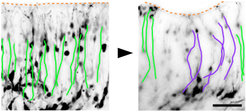
Some evidence suggests that the most recent common mammalian ancestor was gyrencephalic and that several transitions from gyrencephaly to lissencephaly occurred during mammalian evolution. FLRT1/3 expression might have participated in the transition from gyrencephaly to lissencephaly. In the wild-type mouse brain, high levels of FLRT1/3 promote adhesion between neurons resulting in coordinated migration and little lateral dispersion, which favor the formation of smooth cortical layers. Conversely, absence of FLRT1/3 expression reduces adhesion between neurons allowing them to acquire wide dynamic migratory profiles and lateral distribution, which are features characteristic of neurons in the gyrencephalic cortex. Interestingly, the human neocortex expresses much lower levels of FLRT1/3 compared to the lissencephalic mouse neocortex and regions in the ferret neocortex undergoing sulcus formation have lower levels of FLRT1/3 compared to regions developing into a gyrus (Del Toro, Ruff, et al., 2017).
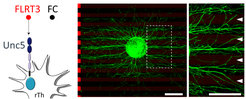
In previous work, we had shown that FLRTs are broadly expressed proteins with the unique property of acting as homophilic cell adhesion molecules and as heterophilic repulsive ligands of Unc5/Netrin receptors (Yamagishi, Hampel, et al., 2011). Using X-ray crystallography, our collaborators at Oxford University and we had revealed the distinct structural bases for FLRT-mediated cell adhesion and repulsion in neurons (Seiradake, del Toro, et al., 2014). We applied this knowledge to elucidate how FLRT functions during cortical development. We showed that FLRTs regulate both the radial migration of pyramidal neurons, as well as their tangential distribution. Mechanistically, radial migration is controlled by repulsive FLRT2-Unc5D interactions, while spatial organization in the tangential axis involves adhesive FLRT-FLRT interactions (Seiradake, del Toro, et al., 2014).
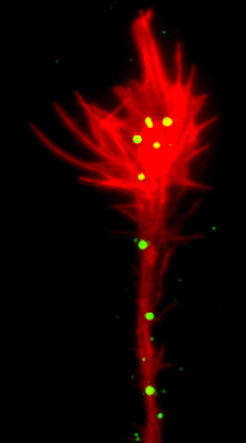
Cell contact–dependent repulsion is an important mechanism to sort and position mixed cell populations, set up tissue boundaries, and guide migrating cells and navigating axons. Ephrin receptor (Eph) tyrosine kinases and their membrane-bound ephrin ligands have emerged as key players in cell sorting, migration, and tissue remodeling. In the developing nervous system, Eph–ephrin signaling commonly generates a repulsive guidance response by inducing axonal growth cone collapse, which are important in, for example, formation of correct retinotopic mapping in the visual system. We are taking a cell biological approach combined with advanced imaging, mass spectrometry, and functional screens to identify the underlying mechanisms of Eph–ephrin-mediated cell repulsion.
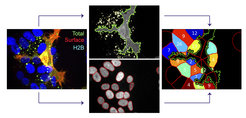
The initial complex of a membrane-anchored repellent cue on the guiding cell and its cognate receptor on the responder cell constitutes a physical tether, which needs to be removed from the cell surface to allow efficient cell detachment. We have previously shown that EphB–ephrinB–mediated repulsion involves trans-endocytosis of the entire receptor–ligand cluster, including parts of the surrounding cell membrane into the opposing cell (Zimmer et al., 2003).
In recent work, we identified an actin-regulating pathway allowing ephrinB+ cells to trans-endocytose EphB receptors from opposing cells (defined as reverse signaling). Live imaging revealed Rac-dependent F-actin enrichment at sites of EphB2 internalization, but not during vesicle trafficking. Systematic depletion of Rho family GTPases and their regulatory proteins identified the Rac subfamily and the Rac-specific guanine nucleotide exchange factor Tiam2 as key components of EphB2 trans-endocytosis, a pathway previously implicated in Eph forward signaling, in which ephrins act in trans as ligands of Eph receptors. We also showed that this pathway is required for EphB2-stimulated contact repulsion. These results support the existence of a conserved pathway for EphB trans-endocytosis that removes the physical tether between cells, thereby enabling cell repulsion (Gaitanos, Koerner, Klein, 2016).
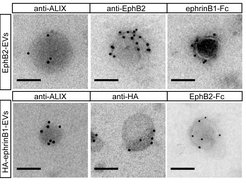
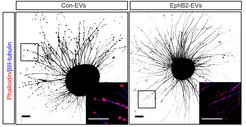
Until recently, it was thought that ephrin-Eph signaling required direct cell contact. However, we reported the cellular release of membranous vesicles known as extracellular vesicles (EVs) or exosomes containing Ephs and ephrins. This raised the possibility that EVs participate in ephrin-Eph signaling independent of direct cell contact. Indeed, we could show that purified EphB2-containing EVs induce ephrinB1 reverse signaling and cause neuronal axon repulsion in a cell biological assay. These results suggest the participation of EphB2+ EVs in neural development and synapse physiology (Gong et al., 2016)